Understanding Your Energy Consumption
Accurately determining your household’s energy usage is crucial for sizing your solar panel system correctly. Overestimating leads to unnecessary costs, while underestimating leaves you with insufficient power. This section details how to assess your average daily energy consumption and provides methods for measuring individual appliance energy use.
Determining your household’s average daily energy usage involves reviewing your electricity bills and analyzing your appliance usage patterns. Your electricity bill provides a historical record of your energy consumption, usually measured in kilowatt-hours (kWh). By examining several months of bills, you can establish an average monthly consumption, which can then be divided by the number of days in the month to arrive at an average daily consumption. This average provides a baseline for your solar panel sizing calculations. However, remember that seasonal variations in energy usage (e.g., higher usage during winter months due to heating) should be considered when making your final assessment.
Measuring Appliance Energy Consumption
Precisely measuring the energy consumption of individual appliances helps refine your overall energy usage estimate. There are several methods to achieve this. Using a Kill-A-Watt meter is a simple and effective approach. This device plugs into a wall outlet and measures the power draw of any appliance plugged into it, providing readings in watts and kilowatt-hours. Alternatively, you can check the appliance’s nameplate, which often displays the wattage. Multiplying the wattage by the hours of daily use gives the daily energy consumption in watt-hours (Wh), which can be converted to kilowatt-hours (kWh) by dividing by 1000. For appliances with varying usage patterns (like refrigerators or air conditioners), observe their usage over several days to obtain a more representative average.
Common Household Appliance Energy Usage
The following table compares the energy usage of common household appliances. Note that these values are estimates, and actual consumption may vary depending on the appliance’s model, age, and usage patterns. Always refer to your appliance’s nameplate for the most accurate wattage information.
Appliance | Wattage (W) | Daily Usage (hours) | Daily Energy Consumption (kWh) |
---|---|---|---|
Refrigerator | 150 | 24 | 3.6 |
Television (LCD) | 100 | 4 | 0.4 |
Laptop | 50 | 2 | 0.1 |
Washing Machine | 500 | 1 | 0.5 |
Dishwasher | 1200 | 1 | 1.2 |
Air Conditioner | 1500 | 6 | 9 |
Electric Oven | 2500 | 1 | 2.5 |
Lighting (LED Bulbs) | 10 | 8 | 0.08 |
Estimating Solar Panel Output
Accurately estimating your solar panel output is crucial for sizing your system correctly. This involves understanding several key factors that influence the efficiency of solar panels and their ability to generate electricity. Overestimating or underestimating can lead to either insufficient power or unnecessary expense.
Several factors significantly impact the efficiency of solar panels and the amount of energy they produce. These include the number of sunlight hours your location receives, the angle at which your panels are positioned relative to the sun, and prevailing weather conditions. Let’s explore each in more detail.
Factors Affecting Solar Panel Efficiency
Sunlight intensity and duration are paramount. The more sunlight your panels receive, the more electricity they will generate. Panel angle significantly affects energy production; optimally angled panels capture more sunlight throughout the day. Finally, weather conditions like cloud cover, rain, and snow reduce sunlight reaching the panels, thus decreasing their output. A sunny day will yield far more energy than a cloudy one. For example, a location with consistently cloudy weather will require a larger solar array to achieve the same energy output as a location with abundant sunshine.
Calculating Peak Sun Hours
Peak sun hours represent the equivalent number of hours the sun shines at its maximum intensity throughout a day. This isn’t simply the number of daylight hours; it accounts for the sun’s angle and intensity. Determining peak sun hours for a specific location requires access to solar irradiance data, often available from online resources or weather services specific to your region. Many online calculators are available; you input your location’s coordinates, and the calculator estimates the peak sun hours based on historical solar data. For example, a location like Yuma, Arizona, known for its abundant sunshine, would have significantly higher peak sun hours than a location in Seattle, Washington.
Impact of Panel Orientation on Energy Production
The orientation of your solar panels directly impacts their energy production. South-facing panels (in the Northern Hemisphere) generally receive the most sunlight throughout the year. However, the optimal angle can vary based on latitude and the time of year. East and west-facing panels can also contribute, but their output will be less than a south-facing installation. The following table illustrates the potential impact of different orientations:
Orientation | Daily Sun Hours (Estimate) | Estimated Energy Output (kWh)* | Efficiency Percentage (relative) |
---|---|---|---|
South-facing (optimal angle) | 6 | 18 | 100% |
East-facing | 3 | 9 | 50% |
West-facing | 3 | 9 | 50% |
North-facing (Northern Hemisphere) | 1 | 3 | 17% |
*These figures are illustrative examples and will vary significantly based on panel size, wattage, and specific location.
Choosing the Right Solar Panel Type
Selecting the appropriate solar panel type is crucial for maximizing your system’s efficiency and lifespan while remaining within your budget. The three primary types—monocrystalline, polycrystalline, and thin-film—each offer unique performance characteristics, influencing their suitability for different applications and individual needs. Understanding these differences will empower you to make an informed decision.
Monocrystalline, Polycrystalline, and Thin-Film Solar Panel Comparison
The choice between monocrystalline, polycrystalline, and thin-film solar panels involves weighing efficiency, cost, and durability. Each technology presents distinct advantages and disadvantages.
- Monocrystalline Silicon: These panels are made from a single, pure silicon crystal, resulting in a dark, uniform appearance. They are known for their high efficiency, typically ranging from 18% to 22%, meaning they convert a larger percentage of sunlight into electricity compared to other types. However, this higher efficiency comes at a premium, making them generally more expensive. Their lifespan is also typically longer, often exceeding 25 years. A potential drawback is their slightly higher susceptibility to shading compared to polycrystalline panels.
- Polycrystalline Silicon: These panels are made from multiple silicon crystals, giving them a characteristic blueish appearance with a speckled texture. They are less efficient than monocrystalline panels, typically ranging from 15% to 17% efficiency. This lower efficiency translates to a lower cost, making them a popular choice for budget-conscious homeowners. Their lifespan is slightly shorter than monocrystalline panels, but still generally sufficient for a long-term investment.
- Thin-Film Solar Panels: These panels are made by depositing a thin layer of photovoltaic material onto a substrate, such as glass or metal. They are known for their flexibility and ability to be integrated into various surfaces. However, they have the lowest efficiency among the three types, typically ranging from 7% to 13%. Their lower cost is often a key advantage, and they can perform well in low-light conditions. Their lifespan is generally comparable to polycrystalline panels.
Advantages and Disadvantages of Different Solar Panel Types
The following table summarizes the key advantages and disadvantages of each solar panel type:
Feature | Monocrystalline | Polycrystalline | Thin-Film |
---|---|---|---|
Efficiency | High (18-22%) | Moderate (15-17%) | Low (7-13%) |
Cost | High | Moderate | Low |
Lifespan | Long (25+ years) | Moderate (20-25 years) | Moderate (20-25 years) |
Appearance | Uniform dark color | Blueish with speckled texture | Variable depending on material and substrate |
Temperature Coefficient | Relatively low | Relatively high | Relatively high |
Note: The actual efficiency and lifespan of solar panels can vary depending on factors such as manufacturing quality, environmental conditions, and maintenance.
Determining System Size and Capacity
Calculating the right solar panel system size involves understanding the interplay between individual panel wattage, the overall system size (in kilowatts), and the total energy your system will produce annually. Essentially, you need to determine how many panels, each with a specific power output, are required to meet your household’s energy needs. This calculation ensures you invest in a system that is both effective and cost-efficient.
The relationship between panel wattage, system size, and energy production is straightforward: Higher wattage panels produce more energy individually, meaning fewer panels are needed for a given system size. System size, measured in kilowatts (kW), directly reflects the total power output capacity of your entire solar array. Total annual energy production is then determined by the system’s size and the amount of sunlight your location receives.
Calculating the Number of Solar Panels
To determine the number of solar panels needed, you’ll use your estimated daily energy consumption (kWh), the average daily sunlight hours in your location, and the wattage of the solar panels you’ve chosen. This calculation considers the variability of sunlight throughout the year and aims to provide a system capable of meeting your energy needs even during periods of less intense sunlight. For example, if your home consumes 20 kWh per day and your chosen panels have a wattage of 300W, and your location receives an average of 4 sun hours per day, the calculation would proceed as follows.
First, calculate the daily energy generation needed from your solar panels:
Daily energy needed / Average daily sun hours = Watts needed per panel
20 kWh/day * 1000 W/kW / 4 sun hours/day = 5000 W/day
Next, determine the number of panels needed:
Watts needed per day / Panel wattage = Number of panels
5000 W / 300 W/panel = 16.67 panels
Since you can’t install a fraction of a panel, you’ll need to round up to 17 panels to ensure sufficient energy generation. This example demonstrates the process; actual calculations will vary depending on your specific energy consumption, panel choice, and sunlight availability. Consult with a solar installer to get a precise calculation tailored to your location and energy requirements.
Step-by-Step Guide for Sizing a Solar Panel System
A systematic approach ensures you choose a solar panel system that optimally meets your needs. This process involves several crucial steps.
- Determine your average daily energy consumption: This involves reviewing your electricity bills over the past year to establish an average daily usage in kilowatt-hours (kWh).
- Assess your location’s solar irradiance: Use online resources or consult with a solar installer to determine the average daily peak sun hours (usually 4-6 hours depending on location and season) for your specific location. This data accounts for variations in sunlight intensity throughout the year.
- Select your solar panels: Choose a panel type based on your budget, space constraints, and efficiency requirements. Note the wattage of the chosen panel. Consider factors like panel efficiency, size, and warranty.
- Calculate the required system size (kW): Multiply your daily energy consumption (kWh) by 1000 (to convert to watts) and divide by the average daily peak sun hours. This will give you the total wattage required. Convert this to kilowatts by dividing by 1000.
- Determine the number of panels: Divide the total wattage required by the wattage of your chosen solar panel to find the number of panels needed. Always round up to the nearest whole number.
- Factor in system losses: Real-world systems experience energy losses due to various factors (e.g., inverter efficiency, wiring losses, shading). Add a safety margin (typically 10-20%) to your calculated number of panels to account for these losses.
- Consult a solar installer: A professional installer can conduct a site assessment, factor in local regulations, and provide a customized system design.
Remember that this is a general guideline. Professional consultation is crucial to ensure the most accurate and efficient system design for your specific circumstances.
Incorporating Energy Storage (Batteries)
Adding a battery storage system to your solar panel setup significantly enhances your ability to utilize the energy generated. Batteries store excess solar energy produced during peak sunlight hours, making it available for use during the evening or on cloudy days, thus maximizing self-sufficiency and reducing reliance on the grid. This section will explore the crucial factors to consider when integrating battery storage into your solar power system.
Battery System Selection Factors
Choosing the right battery system involves careful consideration of several key factors. These factors directly impact the system’s effectiveness, longevity, and overall cost. A well-informed decision ensures optimal performance and return on investment.
Battery Capacity, Lifespan, and Cost
Battery capacity, measured in kilowatt-hours (kWh), determines how much energy the battery can store. A larger capacity allows for longer periods of energy independence, particularly useful in areas with frequent power outages or inconsistent sunlight. Lifespan, typically measured in charge-discharge cycles, indicates how many times the battery can be fully charged and discharged before its capacity significantly degrades. Finally, the cost per kWh reflects the overall financial investment required. Balancing these three factors is essential to finding the most suitable battery system for your needs. For example, a larger capacity battery may have a higher upfront cost but could offer longer-term savings by reducing reliance on grid electricity. Conversely, a smaller, less expensive battery might require more frequent replacements, impacting long-term cost-effectiveness.
Comparison of Battery Technologies
Different battery technologies offer varying performance characteristics. The following table compares common options, highlighting their capacity, lifespan, and cost per kWh. Note that these values can vary based on manufacturer and specific model. It’s crucial to consult current market data for the most up-to-date pricing and specifications.
Battery Type | Capacity (kWh) | Lifespan (cycles) | Cost per kWh |
---|---|---|---|
Lead-Acid (Flooded) | Variable, typically smaller capacities | 300-500 | Relatively low |
Lead-Acid (AGM/Gel) | Variable, typically moderate capacities | 500-800 | Moderate |
Lithium-ion (LFP) | Variable, typically higher capacities | 2000-6000+ | Higher |
Lithium-ion (NMC) | Variable, typically higher capacities | 1000-3000 | High |
Inverter Selection and Sizing
Solar inverters are crucial components of any photovoltaic (PV) system, converting the direct current (DC) electricity generated by solar panels into alternating current (AC) electricity usable in homes and businesses. The correct inverter size significantly impacts the overall efficiency and performance of your solar energy system. An undersized inverter will limit the power output of your solar array, while an oversized inverter may be less efficient and more expensive than necessary.
The process of selecting an appropriately sized inverter involves carefully considering the total DC power output of your solar panel array. This is typically expressed in kilowatts (kW). You need an inverter with a capacity equal to or slightly greater than the peak power output of your panels. It’s generally recommended to choose an inverter with a capacity that’s 10-20% larger than the total DC power of your solar array to account for future expansion or variations in panel output. For example, if your solar array has a peak DC output of 5 kW, you should consider an inverter with a capacity between 5.5 kW and 6 kW. Always consult with a qualified solar installer to ensure the correct sizing for your specific system requirements.
Inverter Types and Specifications
Choosing the right inverter also involves understanding the various types available and their specific features. Different inverters cater to different system needs and budgets.
- String Inverters: These are the most common type, connecting multiple solar panels in series (a string) and converting the combined DC power to AC. They are generally less expensive but offer less flexibility and potentially lower efficiency if one panel in the string is shaded or malfunctions. A typical example might be a 6kW string inverter suitable for a residential rooftop system with multiple south-facing panels.
- Microinverters: Each solar panel has its own microinverter, converting DC to AC individually. This offers greater flexibility, improved energy harvesting (even if some panels are shaded), and enhanced monitoring capabilities. However, they are typically more expensive per kilowatt than string inverters. A home with significant shading might benefit from a system using microinverters, ensuring maximum power output from each panel despite shading conditions.
- Power Optimizers: These devices sit between the solar panels and the inverter, optimizing the performance of individual panels. They offer some of the benefits of microinverters (like improved performance in shaded conditions) at a lower cost than a full microinverter system. This can be a cost-effective alternative to microinverters for systems experiencing partial shading.
- Hybrid Inverters: These inverters combine AC and DC outputs, allowing for the integration of battery storage. They offer the advantage of both immediate solar power usage and the ability to store excess energy for later use. A system utilizing a 5kW hybrid inverter could power a home during the day and also store excess solar energy for nighttime use.
Considering Roof Space and Shading
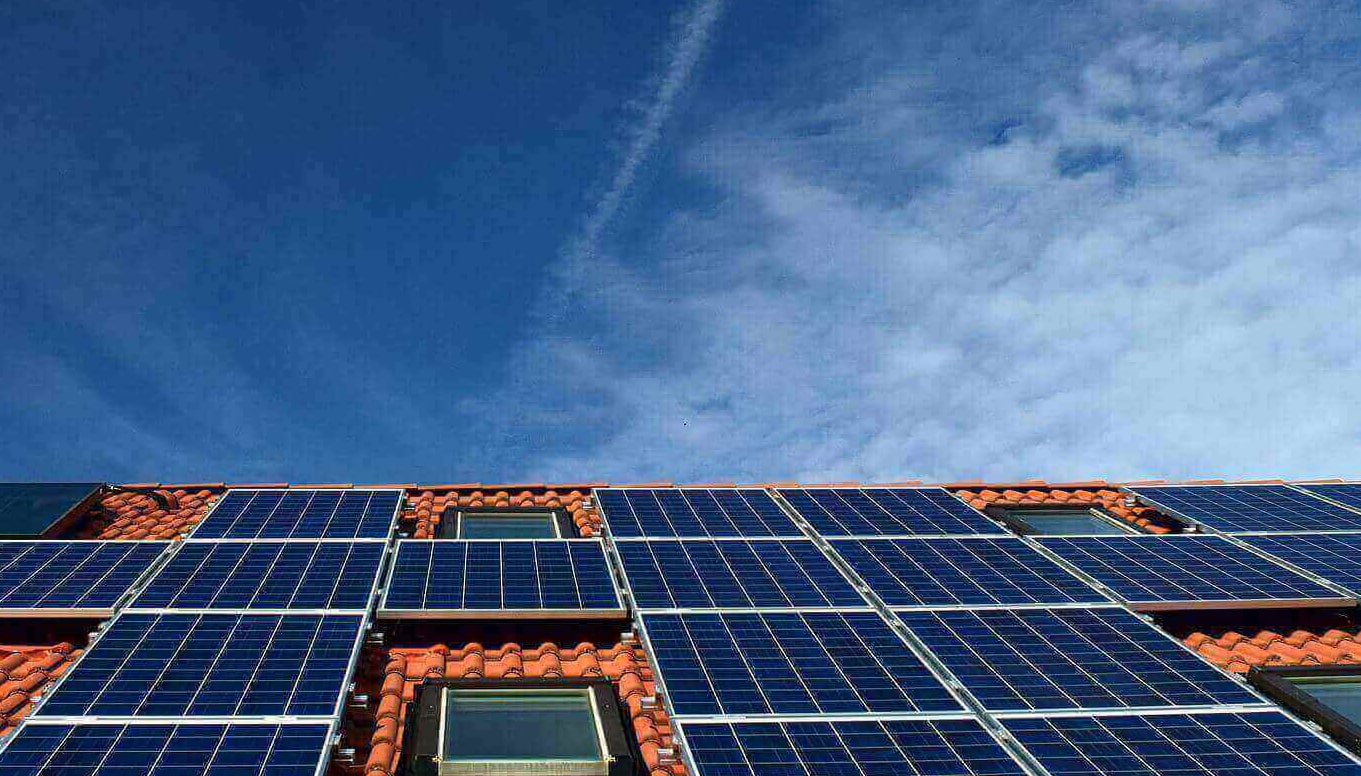
Proper roof assessment and shading mitigation are crucial for maximizing solar panel efficiency and overall system performance. Ignoring these factors can significantly reduce energy production and the return on your investment. This section details how to evaluate your roof’s suitability and address potential shading issues.
Assessing roof suitability involves several key considerations. A thorough evaluation ensures the roof can safely and effectively support a solar panel system.
Roof Structure and Suitability Assessment
The structural integrity of the roof is paramount. This includes considering the roof’s material (e.g., asphalt shingles, tile, metal), its load-bearing capacity, and its overall condition. A qualified solar installer will conduct a detailed inspection to determine if the roof can handle the added weight of the solar panels, mounting hardware, and associated equipment. They will assess the roof’s angle, orientation, and any potential structural weaknesses. For example, a very old roof with significant wear and tear might require repairs or reinforcements before solar panel installation. Similarly, a roof with a very low slope might require specialized mounting systems. The installer will also verify the roof’s ability to withstand the stresses of wind and snow loads, ensuring the system’s long-term stability and safety.
Shading Impact and Mitigation Strategies
Shading, even partial shading, significantly reduces a solar panel’s energy output. Imagine a solar panel as a grid of individual cells; if even one cell is shaded, it can impact the entire panel’s performance, acting like a bottleneck in the electrical flow. This effect is known as the “shading effect.” A single tree, building, or even a chimney casting a shadow on a portion of the array during part of the day will decrease overall energy production. The longer the shading period and the larger the shaded area, the more pronounced this effect will be. For instance, a tree shading a panel for several hours each afternoon will result in a noticeable reduction in daily energy generation compared to a panel in full sun.
Shading Analysis and Mitigation Techniques
Accurate shading analysis is crucial. This typically involves a site visit by a solar installer who will use specialized software or tools to map the shadows cast on the roof throughout the day and year. Mitigation strategies depend on the nature and extent of the shading. Trimming overhanging branches is a common solution for minor shading issues. Relocating the panels to avoid shaded areas might be necessary in more severe cases. More complex solutions, such as installing microinverters (which allow individual panels to operate independently), can also improve performance in partially shaded conditions. For example, a system with microinverters will continue to produce energy from unshaded panels even if some are shaded, unlike a traditional string inverter system where shading on one panel can significantly impact the entire string’s output. A thorough shading analysis allows for the most effective mitigation strategy to be chosen, maximizing energy production and ensuring a cost-effective solar installation.
Accounting for System Losses
Designing a solar panel system requires careful consideration of energy losses that occur throughout the system. These losses reduce the overall efficiency and the amount of usable energy generated. Understanding and accounting for these losses is crucial to accurately sizing your system and ensuring it meets your energy needs. Failing to account for these losses can lead to a system that underperforms expectations.
Several factors contribute to energy losses in a solar power system. These losses are not insignificant and can cumulatively reduce the system’s output by 10-15%, or even more in some cases. Accurate estimation of these losses is vital for ensuring your system is appropriately sized to meet your energy demands.
Wiring Losses
Wiring losses occur due to the resistance of the wires connecting the solar panels, the charge controller, the inverter, and ultimately, your home’s electrical system. Longer wire runs and smaller gauge wires lead to increased resistance and higher energy losses. These losses are directly proportional to the square of the current flowing through the wires and the resistance of the wires themselves. For example, a system with long runs of thin wire might experience a 2-3% loss due to resistance alone. Using larger gauge wire and minimizing wire length can significantly mitigate this loss. Proper wire sizing and installation are essential for minimizing these losses and ensuring efficient energy transfer.
Inverter Losses
Inverters convert the direct current (DC) electricity produced by the solar panels into alternating current (AC) electricity usable in your home. Inverters are not 100% efficient; they typically have losses ranging from 3-5%. These losses are largely due to heat generated during the conversion process. Higher-efficiency inverters can minimize these losses, and selecting a high-efficiency inverter is a key aspect of system design. For instance, an inverter with 95% efficiency will still result in a 5% loss of the DC power generated by the panels.
Temperature Losses
Solar panels generate less electricity in hot temperatures. The efficiency of solar panels decreases as their temperature increases. This temperature-dependent loss can range from 0.3% to 0.5% per degree Celsius above the standard test condition temperature (STC) of 25°C. For example, if the panel temperature is 45°C, the potential loss could be around 6-10%. Proper panel orientation, ventilation, and shading can help mitigate temperature-related losses.
Shading Losses
Partial shading of even a single solar panel can significantly impact the overall system output. A shaded cell in a panel can act as a current bottleneck, reducing the power output of the entire string of panels connected in series. Careful consideration of shading from trees, buildings, or other structures is crucial during system design. Employing techniques such as microinverters or power optimizers can help mitigate shading losses by allowing individual panels to operate independently.
Other Losses
Other minor losses can also occur, such as those due to soiling (dust, dirt, bird droppings) on the panels and mismatch losses between panels. Regular cleaning of the panels can minimize soiling losses, while careful selection and matching of panels can minimize mismatch losses. These combined losses can represent another 1-3% reduction in overall system output.
Estimating and Incorporating Losses
To accurately estimate total system losses, it is recommended to add the individual loss percentages. For instance, if you estimate wiring losses at 2%, inverter losses at 4%, temperature losses at 8%, shading losses at 2%, and other losses at 3%, the total estimated loss would be 19%. This 19% loss should then be factored into the system design by increasing the initial solar panel capacity to compensate for these losses. Therefore, if your energy needs require a 5kW system without considering losses, you would need a system with a capacity of approximately 6 kW (5 kW / (1-0.19)) to account for the 19% loss. This ensures the system delivers the required energy output despite the various inefficiencies.
Minimizing Energy Losses
Minimizing energy losses requires a holistic approach during the design and installation phases. This includes:
- Using appropriately sized and high-quality wiring.
- Selecting high-efficiency inverters.
- Optimizing panel placement to minimize shading and temperature effects.
- Regular cleaning of the panels to reduce soiling losses.
- Implementing advanced technologies such as microinverters or power optimizers to mitigate shading and mismatch losses.
Local Regulations and Permits
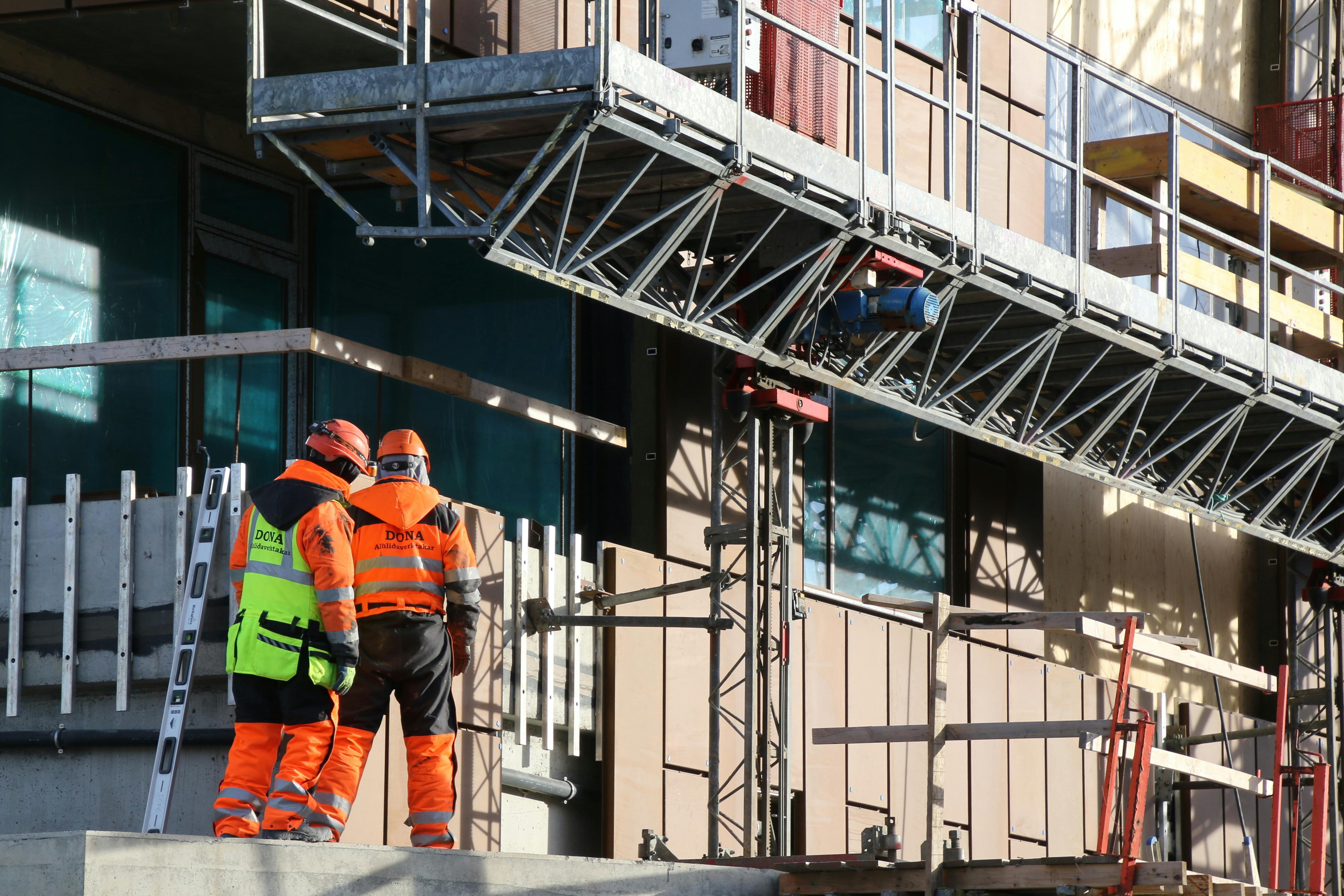
Successfully installing a solar panel system requires navigating the legal landscape of local regulations and obtaining the necessary permits. This process ensures your system is installed safely and complies with all building codes and environmental standards, preventing potential future issues. Understanding these regulations is crucial for a smooth and legally sound solar energy project.
Local regulations governing solar panel installations vary significantly depending on your location. These regulations often address aspects such as system size, placement, safety requirements, and interconnection with the electrical grid. Failing to comply with these regulations can lead to delays, fines, and even the forced removal of your solar panel system. Therefore, proactive research and adherence to the permitting process are essential.
Finding Local Regulations and Guidelines
Locating the relevant regulations typically involves contacting your local government agencies. This often includes your city or county building department, planning department, and potentially your local utility company. Many municipalities have websites dedicated to building codes and permits, where you may find specific guidelines for renewable energy systems. In some cases, your local utility company may provide additional resources or even offer guidance on the permitting process. It is also helpful to search online for your city or county name plus “solar permitting” or “solar regulations” to find specific documents and contact information.
The Solar Panel Permitting Process
The permitting process generally follows a structured sequence of steps, although specifics may vary depending on your location. It’s advisable to contact your local authorities early in the planning stages to clarify the exact requirements and timelines.
A typical permitting process might involve these steps:
- Initial Inquiry and Application: Begin by contacting your local permitting authority to inquire about the specific requirements for solar panel installations in your area. Obtain the necessary application forms and gather all the required documentation, which may include architectural drawings, system specifications, and proof of ownership.
- Application Review and Plan Check: Submit your completed application and supporting documents. The permitting authority will review your application to ensure compliance with all relevant codes and regulations. This review may include a plan check, where a building inspector examines your plans to verify the proposed system’s safety and compliance.
- Permit Issuance: Once your application and plans are approved, the permitting authority will issue a permit authorizing the installation of your solar panel system. This permit typically has an expiration date, so it’s important to start installation within the specified timeframe.
- Inspection: During and after the installation, you may be required to schedule inspections with the building inspector to verify compliance with the approved plans and building codes. These inspections ensure the proper installation of the system and adherence to safety standards.
- Final Approval and Connection: Once all inspections are successfully completed, you will receive final approval. You can then connect your solar panel system to the electrical grid (if applicable) and begin generating clean energy.
Resources for Finding Local Regulations
Beyond contacting local government agencies directly, several online resources can assist in locating relevant regulations and guidelines. These resources often include state-level agencies focused on renewable energy, national organizations dedicated to solar energy, and online databases of building codes. It’s essential to verify the information found online with your local authorities to ensure accuracy and compliance with the most up-to-date regulations. Many solar installers also have experience navigating local permitting processes and can assist you in this step.
FAQ Summary
What is the average lifespan of a solar panel?
Most solar panels have a lifespan of 25-30 years, though they continue to produce power beyond that, albeit at a reduced efficiency.
How often should I have my solar panel system inspected?
Annual inspections are recommended to identify and address potential issues, ensuring optimal performance and longevity.
What are net metering programs, and how do they work?
Net metering allows you to sell excess solar energy generated by your system back to the power grid, reducing your overall electricity bill.
Can I install solar panels myself?
While DIY installation is possible, it’s generally recommended to hire a qualified installer to ensure safety, efficiency, and compliance with regulations.