Solar Panel Functionality in Low Light
Solar panels continue to generate electricity even on cloudy or rainy days, albeit at a reduced rate compared to bright sunshine. This is because they don’t rely on direct sunlight alone; they can also harness diffuse sunlight – the sunlight scattered by clouds and atmospheric particles. Understanding how this works is key to appreciating the consistent energy production of solar panels even under less-than-ideal weather conditions.
Solar panels generate electricity through the photovoltaic effect. When sunlight strikes a solar cell, photons (light particles) excite electrons in the semiconductor material (typically silicon). This excitation causes electrons to break free from their atoms, creating a flow of electrons – an electric current. Even on a cloudy day, while the intensity of direct sunlight is reduced, there’s still a significant amount of diffuse sunlight reaching the Earth’s surface. This diffuse light still contains photons that can excite electrons in the solar cells, albeit at a lower rate than direct sunlight. The physics remains the same; the quantity of photons, and thus the current generated, is simply less.
Energy Output Comparison: Sunny vs. Cloudy Days
The energy output of solar panels varies significantly depending on the intensity and duration of sunlight. On a bright, sunny day with minimal cloud cover, a solar panel can achieve its peak power output. However, on a cloudy day, the output is considerably lower. The reduction in output is directly proportional to the decrease in sunlight intensity. For example, if the sunlight intensity is reduced by 50% due to cloud cover, the solar panel’s power output will also be approximately 50% lower. This reduction isn’t linear, however; factors like cloud density and the type of clouds influence the precise reduction. A heavily overcast day might yield only 10-20% of peak output, while a partly cloudy day might produce 50-70%. Real-world examples include studies that have shown consistent energy production even during periods of prolonged cloud cover, though the overall yield over a month is naturally lower than in a sunnier month.
Solar Cell Types and Low-Light Performance
Different types of solar cells exhibit varying levels of efficiency in low-light conditions. Traditional crystalline silicon solar cells, while dominant in the market, are relatively less efficient in diffuse light compared to some newer technologies. Thin-film solar cells, such as amorphous silicon, cadmium telluride (CdTe), and copper indium gallium selenide (CIGS), generally perform better in low-light conditions due to their ability to absorb a broader spectrum of light. This is because their structure allows them to capture more of the scattered photons present in diffuse sunlight. However, thin-film cells typically have lower overall efficiency compared to crystalline silicon cells under optimal sunlight conditions. The choice of solar cell technology depends on a balance between low-light performance and overall efficiency. For example, a rooftop installation in a consistently cloudy region might benefit more from thin-film cells, while a sunny location might prioritize the higher peak efficiency of crystalline silicon.
Impact of Rain on Solar Panel Performance
Rain, while seemingly detrimental to solar energy production, has a more nuanced effect on solar panel performance than simply blocking sunlight. While heavy downpours will naturally reduce energy output, the impact is less severe than one might initially assume, and the panels themselves are designed to withstand the elements. Understanding the interplay between rain and solar panel efficiency is key to maximizing energy generation even in wet climates.
Rain’s effect on solar panel efficiency is primarily a matter of light absorption. Water droplets on the surface of a panel can scatter and reflect incoming sunlight, reducing the amount of light that reaches the photovoltaic cells. This scattering effect is more pronounced with larger droplets and at certain angles of incidence. However, this reduction in efficiency is generally temporary and minimal, particularly with modern panels designed with anti-reflective coatings. The amount of energy loss depends on several factors including the intensity of the rainfall, the duration, and the angle of the sun.
Water Droplet Effects on Light Absorption
The interaction between water droplets and sunlight is complex. While some light is reflected, a significant portion is still transmitted through the droplets and reaches the solar cells. The refractive index of water causes the light to bend as it passes through the droplets, potentially leading to a slight redistribution of light intensity across the panel’s surface. This effect is often negligible compared to the overall energy production, especially in moderate rainfall. However, in extremely heavy downpours, the combined effect of reduced light penetration and light scattering could lead to a more noticeable decrease in energy output. Imagine a clear glass of water – you can still see through it, even if the surface is slightly distorted by ripples or droplets. Similarly, rain affects the amount of light hitting the cells, but doesn’t entirely block it.
Potential Damage from Prolonged Rain Exposure
While solar panels are designed to withstand harsh weather conditions, including prolonged exposure to rain, there are potential concerns. Prolonged exposure to moisture can increase the risk of corrosion on the panel’s metal components, especially the frame and wiring. Furthermore, while rare, extremely heavy and persistent rain could potentially lead to water ingress into the panel’s internal components, causing damage to the photovoltaic cells. This is more likely if the panel’s sealant has been compromised, for example, through physical damage or age. Regular inspection and maintenance are crucial to mitigate these risks.
Best Practices for Maintaining Solar Panels in Rainy Climates
Regular cleaning is essential to maintain optimal performance in rainy climates. Accumulated dirt, debris, and bird droppings can significantly reduce efficiency, even more so when combined with the effects of rain. A simple rinse with water can effectively remove most contaminants. However, it’s important to use soft water and avoid harsh chemicals that could damage the panel’s surface. Additionally, periodic inspections should be conducted to check for any signs of damage, such as cracks, corrosion, or loose wiring. Early detection and repair of any issues can prevent more extensive and costly repairs in the future. Proper installation, including ensuring appropriate tilt and orientation, can also help minimize water accumulation and improve drainage, thus reducing the risk of prolonged water exposure.
Energy Production in Different Weather Conditions
Solar panel energy production is directly influenced by the amount of sunlight available. While optimal performance occurs under bright, sunny skies, panels still generate electricity, albeit at reduced levels, even on cloudy or rainy days. Understanding this variability is crucial for accurate energy estimations and system planning.
The following sections detail how solar panel energy output changes across various weather conditions, providing a clearer picture of their performance under less-than-ideal circumstances.
Solar Energy Output Across Weather Conditions
The table below illustrates the approximate energy generation of a typical solar panel system under different weather scenarios. These figures are estimates and can vary based on factors like panel type, angle of installation, and cloud density. For example, a heavily overcast day will produce significantly less energy than a day with scattered clouds.
Weather Condition | Average Sunlight Intensity | Estimated Energy Output Percentage | Notes |
---|---|---|---|
Sunny (clear skies) | High (1000 W/m²) | 100% | Optimal conditions for maximum energy production. |
Partly Cloudy | Medium (500-700 W/m²) | 50-70% | Energy output fluctuates depending on cloud cover and sunlight penetration. |
Cloudy (Overcast) | Low (100-300 W/m²) | 10-30% | Significant reduction in energy output; still some energy generation. |
Rainy (Overcast) | Very Low (50-150 W/m²) | 5-15% | Reduced sunlight penetration due to cloud cover and rain; energy production is minimal. |
Relationship Between Cloud Cover and Solar Panel Output
The relationship between cloud cover and solar panel output can be visualized using a graph. Imagine a line graph where the x-axis represents cloud cover percentage (from 0% – clear skies to 100% – completely overcast) and the y-axis represents the percentage of energy output relative to peak sunlight conditions (100%).
The graph would show a generally downward sloping curve. At 0% cloud cover, the energy output would be at 100%. As cloud cover increases, the energy output would gradually decrease, initially at a slower rate and then more steeply as the sky becomes increasingly overcast. The curve wouldn’t be perfectly linear; a sudden increase in cloud density would cause a more significant drop in energy production than a gradual increase. Even with 100% cloud cover, a small amount of diffused light still penetrates, resulting in a small, non-zero energy output.
Impact of Weather on Solar Energy Production
The following points summarize how different weather conditions affect the energy production of solar panels:
- Sunny weather: Provides optimal conditions for maximum energy generation. A sunny day with minimal cloud cover will yield the highest energy output.
- Cloudy weather: Reduces energy output significantly, but panels still produce some electricity. The amount of energy generated depends on the cloud density and type of clouds.
- Rainy weather: Further reduces energy output due to reduced sunlight penetration and the potential for water accumulation on the panel surface. However, rain typically washes away dust and dirt, improving long-term panel efficiency.
- Seasonal variations: Energy production is also influenced by the angle of the sun, which varies throughout the year. Solar panels generally produce more energy during the summer months when the sun is higher in the sky.
Solar Panel Design and Materials
Solar panel design and the materials used significantly influence their performance across diverse weather conditions. Understanding these factors is crucial for optimizing energy production and ensuring long-term efficiency. The interplay between materials and design features determines how effectively a panel converts sunlight into electricity, even under less-than-ideal conditions like cloud cover or rain.
The efficiency of solar panels in various weather conditions is largely determined by the materials used in their construction. These materials work together to capture, convert, and transmit energy.
Solar Panel Materials and Their Impact on Performance
Solar panels primarily consist of silicon, a semiconductor material that forms the photovoltaic cells. Different types of silicon, such as monocrystalline, polycrystalline, and amorphous silicon, offer varying levels of efficiency. Monocrystalline silicon, known for its dark black appearance, is the most efficient but also the most expensive. Polycrystalline silicon, with its characteristic blueish appearance, offers a good balance between efficiency and cost. Amorphous silicon, a thin-film technology, is less efficient but more flexible and suitable for certain applications. The choice of silicon type influences the panel’s ability to generate power in low-light conditions; monocrystalline silicon generally performs better. In addition to silicon, solar panels incorporate other materials such as glass (for protection), ethylene propylene diene monomer (EPDM) rubber (for sealing and weatherproofing), and aluminum (for framing). The glass layer protects the cells from environmental factors such as rain, snow, and hail, minimizing damage and ensuring consistent performance. The EPDM rubber creates a durable and weather-resistant seal, preventing moisture from entering the panel and damaging the internal components. The aluminum frame provides structural support and enhances the panel’s durability. The performance of each material impacts the overall panel efficiency in diverse weather conditions. For instance, the glass’s ability to transmit light is critical, while the EPDM’s weather resistance is crucial for maintaining panel integrity during storms.
Anti-Reflective Coatings and Low-Light Efficiency
Anti-reflective coatings are applied to the surface of solar cells to minimize light reflection and maximize light absorption. These coatings, often composed of silicon nitride or titanium dioxide, reduce the amount of light that bounces off the panel’s surface, increasing the amount of light available for conversion into electricity. This is particularly beneficial in cloudy or rainy weather, where light intensity is lower. A reduction in reflection translates directly to increased energy generation, even in low-light conditions. For example, a panel with a high-quality anti-reflective coating might generate 5-10% more energy compared to a panel without such a coating under cloudy skies.
Design Features for Maximizing Energy Capture in Cloudy or Rainy Weather
Several design features enhance solar panel performance in cloudy or rainy weather. These include:
* Optimized cell arrangement: Efficient cell arrangement minimizes shading effects and maximizes light absorption.
* Improved light trapping: Specific cell designs and surface textures can trap and redirect light, improving efficiency in diffuse light conditions.
* High-transparency encapsulants: The material encapsulating the solar cells should allow maximum light transmission.
* Durable construction: Robust construction ensures the panel can withstand the harsh weather conditions without performance degradation.
Comparison of Solar Panel Designs and Climate Suitability
Different solar panel designs cater to specific climate needs. For example, thin-film solar panels, due to their flexibility, are well-suited for integration into building materials in areas with frequent high winds or hail. Monocrystalline panels, while more expensive, offer higher efficiency and may be preferred in areas with limited sunlight or significant cloud cover. Polycrystalline panels represent a good compromise between cost and performance, making them suitable for a wide range of climates. The choice of panel type should consider the local climate, energy needs, and budget. For instance, in a region with consistently high solar irradiance, a polycrystalline panel might be sufficient, while in a region with frequent cloud cover, a monocrystalline panel might be a more appropriate choice to maximize energy production.
The Role of Sunlight Angle
The angle at which sunlight strikes a solar panel significantly impacts its energy production. A more direct angle, where the sun’s rays hit the panel perpendicularly, results in higher energy generation compared to an oblique angle where the rays strike at a shallower angle. This is because a perpendicular angle maximizes the surface area of the panel exposed to the sun’s rays, allowing for the absorption of more solar energy.
The impact of low sun angles during cloudy or rainy periods is substantial. Clouds and rain reduce the intensity of sunlight reaching the earth’s surface. Simultaneously, low sun angles, common during dawn, dusk, and winter months, further diminish the amount of sunlight striking the panel. This combination results in significantly reduced energy output from solar panels. The scattered nature of light during cloudy conditions also reduces the effectiveness of solar panel absorption.
Sun Angle and Energy Generation
The following diagram illustrates the relationship between sun angle and energy generation. Imagine a square representing a solar panel. Draw a line representing a sun ray hitting the panel at a 90-degree angle (perpendicular). Label this as “Optimal Angle (90°)”. Next, draw another line representing a sun ray hitting the panel at a 45-degree angle. Label this as “Oblique Angle (45°)”. Finally, draw a third line representing a sun ray hitting the panel at a very shallow angle, perhaps 15 degrees. Label this “Low Angle (15°)”. Above each ray, draw a smaller square representing the amount of energy captured. The square above the 90-degree ray should be the largest, indicating maximum energy capture. The square above the 45-degree ray should be smaller, and the square above the 15-degree ray should be the smallest, reflecting significantly reduced energy capture. This visually demonstrates how a more direct sun angle leads to greater energy generation. The size difference between the squares directly correlates to the relative energy generated at each angle.
Optimizing Solar Panel Positioning
Optimizing solar panel positioning is crucial for maximizing energy capture, especially in less-than-ideal weather. Proper orientation and tilt angle can significantly mitigate the negative impacts of low sun angles. In regions with predominantly sunny weather, panels are usually positioned to face the equator (south in the northern hemisphere, north in the southern hemisphere) at an angle roughly equal to the local latitude. This ensures optimal sunlight exposure throughout the year. However, during cloudy or rainy periods, even this optimal positioning can only partially offset the reduction in sunlight intensity. Consider a scenario where a solar panel system is installed in a location with frequent overcast days. By slightly adjusting the tilt angle of the panels throughout the year, to follow the changing sun path, homeowners can improve energy capture during winter months when the sun is lower in the sky. This strategy, although not eliminating the effects of low light conditions, helps maximize energy production despite less-than-ideal weather.
Energy Storage Solutions
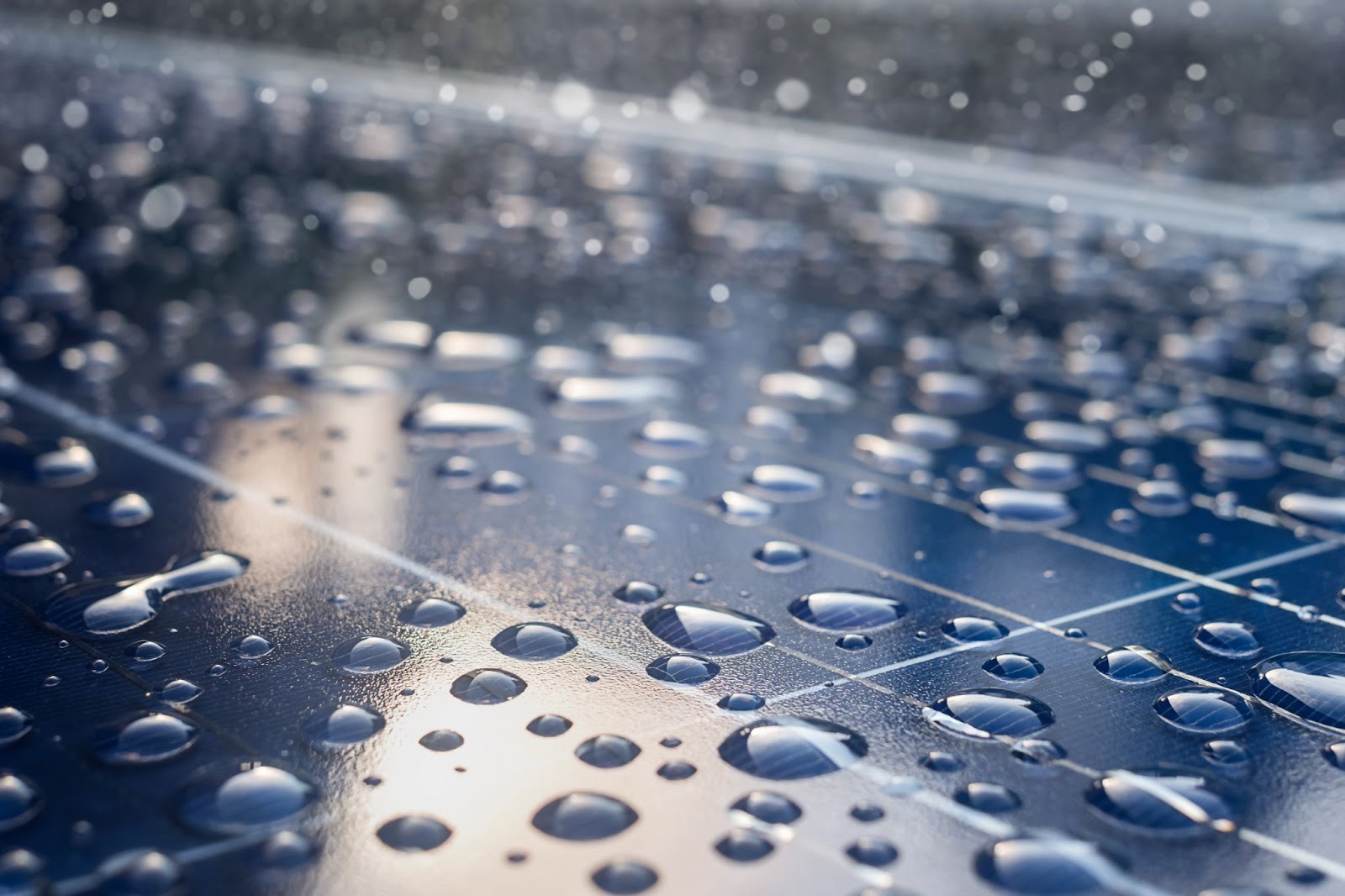
In areas with frequent cloudy or rainy weather, the intermittent nature of solar energy production can significantly impact a household’s reliance on solar power. Battery storage systems bridge this gap, ensuring a consistent supply of electricity even when solar panels aren’t generating at peak capacity. They act as a buffer, storing excess energy generated during sunny periods for use during periods of low sunlight. This significantly increases the self-sufficiency and reliability of a home solar power system.
Battery storage systems are crucial for maximizing the benefits of solar panels, particularly in regions with less consistent sunlight. They reduce reliance on the grid, lowering electricity bills and minimizing carbon footprint. The choice of battery technology depends on factors like budget, energy needs, and available space.
Battery Technologies for Solar Energy Storage
Several battery technologies are suitable for storing solar energy. Each offers a unique combination of advantages and disadvantages concerning cost, lifespan, energy density, and environmental impact.
- Lithium-ion batteries: Currently the most popular choice for home solar energy storage, lithium-ion batteries offer high energy density, meaning they can store a significant amount of energy in a relatively small space. They also have a relatively long lifespan and a fast charge/discharge rate. However, they can be expensive compared to other options and have safety concerns related to fire risk if not properly managed. Examples include Tesla Powerwall and LG Chem RESU.
- Lead-acid batteries: A more mature technology, lead-acid batteries are generally less expensive than lithium-ion batteries. They are also well-understood and readily available. However, they have a shorter lifespan, lower energy density, and require more maintenance compared to lithium-ion batteries. They are less efficient in terms of energy storage capacity per unit volume or weight.
- Flow batteries: Flow batteries offer a longer lifespan and greater scalability than lithium-ion batteries. They are also less susceptible to degradation from frequent charge-discharge cycles. However, they typically have a lower energy density and are currently more expensive than other options. They are often used in large-scale energy storage projects.
Advantages and Disadvantages of Battery Storage with Solar Panels
The decision to incorporate battery storage into a home solar system involves weighing several factors.
- Advantages: Increased energy independence, reduced reliance on the grid, lower electricity bills, backup power during outages, improved energy efficiency by using excess solar energy, and reduced carbon footprint.
- Disadvantages: High initial investment cost, limited lifespan requiring eventual replacement, potential safety hazards (especially with lithium-ion batteries), and the need for regular maintenance.
Cost-Effectiveness of Different Battery Storage Options
The cost-effectiveness of different battery storage options depends on several factors, including battery capacity, technology, installation costs, and government incentives. Generally, lithium-ion batteries offer the best balance of performance and lifespan, although their high upfront cost can be a barrier. Lead-acid batteries are less expensive initially but have a shorter lifespan, potentially making them less cost-effective in the long run. Flow batteries, while offering long lifespans, are currently the most expensive option, making them suitable only for large-scale applications or situations where extremely long lifespans are paramount. The total cost of ownership, factoring in replacement costs over the lifetime of the system, should be carefully considered when comparing different options. For example, a 10 kWh lithium-ion battery system might cost significantly more upfront than a comparable lead-acid system, but its longer lifespan and higher efficiency could result in lower long-term costs. Government incentives, such as tax credits or rebates, can also significantly influence the overall cost-effectiveness of a battery storage system.
Grid Integration and Power Consumption
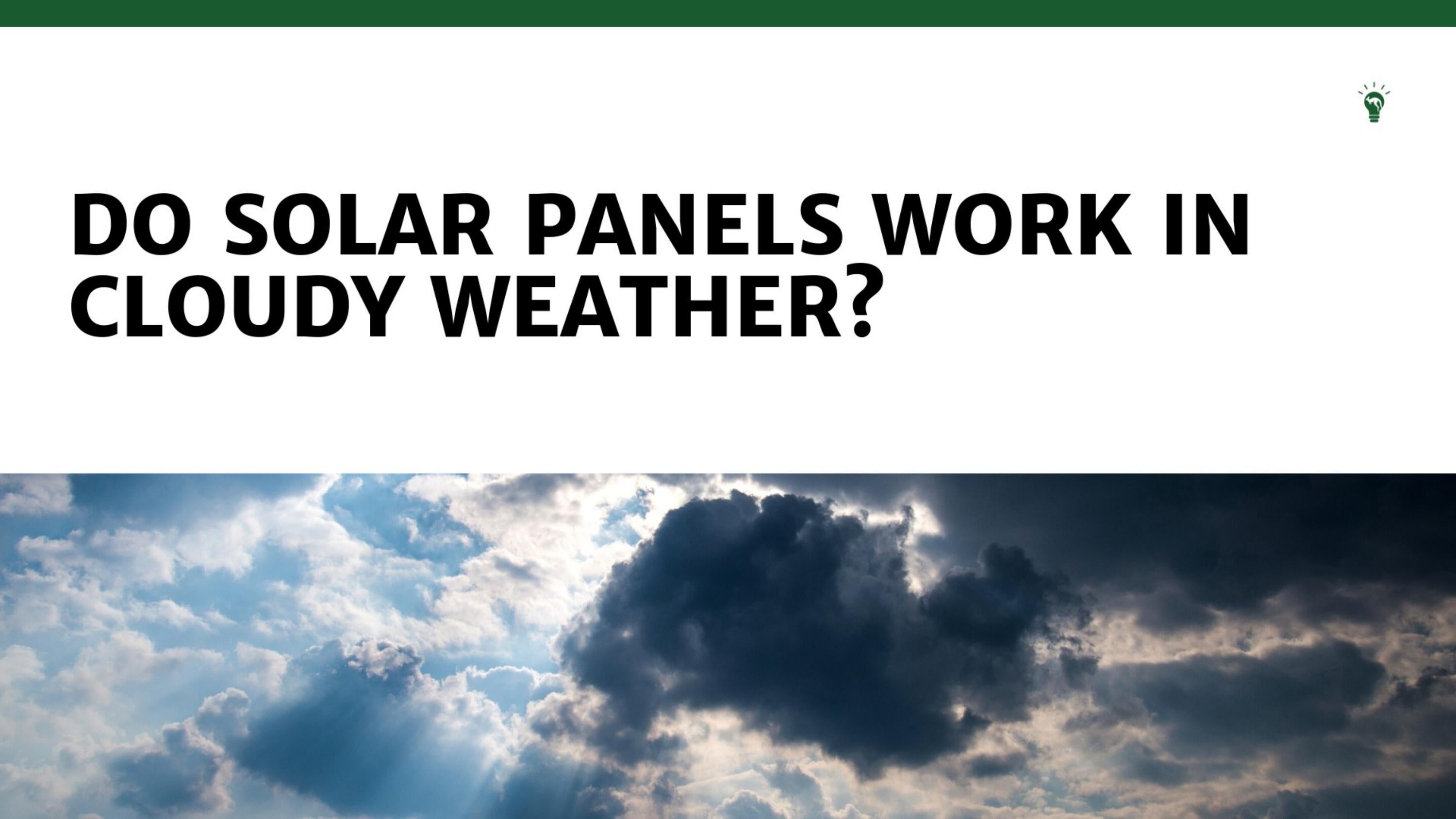
Solar panel systems are integrated into the electricity grid to allow homeowners and businesses to utilize the clean energy they generate while also contributing to the overall power supply. This integration involves several key components and processes that ensure safe and efficient energy flow, both when the sun is shining brightly and when conditions are less favorable.
The integration of solar panels affects energy consumption primarily during periods of low solar output, such as cloudy days or nighttime. When solar energy production decreases, the household or business will rely more heavily on electricity drawn from the grid. The amount of reliance will depend on the size of the solar panel system relative to energy consumption. Smart grid technologies and energy management strategies are crucial in mitigating potential disruptions and optimizing energy use during these periods.
Grid Integration Methods
Solar panel systems are typically connected to the grid through an inverter, which converts the direct current (DC) electricity produced by the panels into alternating current (AC) electricity compatible with household appliances and the grid. This connection can be done through a variety of methods, including a single-phase system for smaller installations and a three-phase system for larger ones. The specific connection method is determined by the size of the system and the requirements of the local grid infrastructure. Safety mechanisms are incorporated to ensure the system disconnects from the grid automatically in case of power outages to prevent accidents involving utility workers.
Smart Inverter Functionality
Smart inverters play a crucial role in managing energy flow between the solar panels, the household, and the grid. They monitor solar energy production, household energy consumption, and grid conditions in real-time. This allows them to optimize energy distribution, maximizing the use of solar energy and minimizing reliance on the grid. Furthermore, many smart inverters offer features such as remote monitoring and control, enabling users to track their energy production and consumption patterns. For instance, a smart inverter might prioritize using solar power for immediate household needs and send excess energy to the grid during peak production periods. During periods of low solar output, the smart inverter seamlessly switches to drawing power from the grid, ensuring a continuous supply of electricity.
Net Metering and Variable Weather
Net metering is a billing mechanism that credits homeowners or businesses for the excess solar energy they feed into the grid. This essentially allows them to “sell” their excess solar power back to the utility company at the retail rate. During periods of variable weather, net metering helps to balance out energy production and consumption. For example, on a sunny day, a household might generate more solar energy than it consumes, receiving credit on their electricity bill. On a cloudy day, when solar production is lower, the household will draw more power from the grid, using the accumulated credit from previous sunny days to offset the cost. This system encourages the adoption of solar energy by mitigating the risks associated with fluctuating solar output. The specific net metering policies vary by region and utility provider, but the core principle remains consistent: it offers a fair compensation mechanism for solar energy generation, even under variable weather conditions.
Long-Term Performance and Maintenance
Solar panels are designed for long-term operation, typically lasting 25-30 years or more. However, their performance and lifespan are significantly influenced by environmental factors, particularly in regions with diverse weather patterns including heavy rainfall, strong winds, and extreme temperatures. Understanding the long-term performance characteristics and implementing a proactive maintenance schedule are crucial for maximizing energy production and extending the lifespan of your solar panel system.
The long-term performance of solar panels is influenced by several factors, including the quality of the materials used in their construction, the efficiency of the panel design, and the environmental conditions they are exposed to. While the initial energy output might be high, it gradually decreases over time, a phenomenon known as degradation. This degradation rate varies depending on factors like cell type, manufacturing quality, and environmental exposure. For example, panels in consistently sunny climates might experience slower degradation compared to those in areas with frequent hailstorms or heavy snowfall. Regular maintenance, such as cleaning, can mitigate the impact of environmental factors and help maintain optimal performance levels.
Impact of Weather on Long-Term Panel Performance
Different weather conditions affect solar panel performance in various ways. Consistent exposure to high temperatures can lead to increased degradation rates, while prolonged periods of extreme cold can reduce efficiency. Heavy rainfall, while not directly damaging to the panels themselves (if properly installed), can reduce energy production due to soiling and shading. Strong winds, especially when combined with hail or debris, pose a significant risk of physical damage, potentially leading to cracks or broken cells. Coastal areas are prone to salt spray corrosion which can affect the panel’s longevity. Regular inspections are vital to identify any early signs of damage or degradation, allowing for timely repairs or replacements.
Regular Cleaning and Maintenance in Rainy Areas
In rainy areas, regular cleaning is essential to maintain optimal solar panel performance. While rain can naturally wash away some dirt and debris, accumulated dust, bird droppings, and pollen can significantly reduce energy output. The frequency of cleaning depends on the local environment; areas with higher levels of air pollution may require more frequent cleaning than those with cleaner air. A simple cleaning using water and a soft brush or sponge is usually sufficient. Pressure washing should be avoided as it can damage the panels. A professional cleaning service might be necessary for large systems or difficult-to-reach areas.
Potential Problems Due to Rain and Wind Exposure
Prolonged exposure to rain can lead to issues such as corrosion of electrical connections and mounting hardware. Wind can cause physical damage, especially to poorly installed systems. Loose connections can result in power outages or even fire hazards. Hailstorms can cause significant damage to the solar panels themselves, leading to cracks, broken cells, and reduced efficiency. Regular inspections are crucial to identify and address these potential problems before they escalate. Proper installation using high-quality materials and techniques is vital to minimize these risks. Consider using robust mounting systems designed to withstand high winds and potential hail impact.
Preventative Maintenance Schedule
A preventative maintenance schedule should be tailored to the specific climatic conditions and the size and complexity of the solar panel system. However, a general guideline might include:
- Visual Inspection: Monthly inspection for any visible damage, loose connections, or debris accumulation.
- Cleaning: Quarterly cleaning, more frequently in areas with high pollution or dust levels. Consider professional cleaning annually.
- Performance Monitoring: Regular monitoring of energy production to identify any significant drops in output, which could indicate a problem.
- Professional Inspection: Annual professional inspection and maintenance by a qualified technician to check for any electrical faults, loose connections, or signs of degradation. This is particularly important in areas with extreme weather conditions.
Environmental Impact and Sustainability
Solar energy offers a compelling alternative to fossil fuels, presenting a significantly lower environmental impact and contributing to a more sustainable energy future. The production and use of solar panels, however, involve various stages with their own environmental considerations. A comprehensive assessment requires a balanced view of both the advantages and potential drawbacks.
The environmental impact of solar energy production is primarily associated with the manufacturing process of solar panels. This includes the extraction and processing of raw materials like silicon, which can be energy-intensive and generate waste. Furthermore, the production of certain components may involve the use of hazardous chemicals. However, the environmental burden associated with solar panel manufacturing is decreasing as technology advances and more sustainable practices are adopted. The lifecycle assessment of solar panels shows that their overall environmental impact is considerably lower than that of fossil fuel-based energy sources.
Carbon Footprint Comparison
The carbon footprint of solar energy is significantly smaller than that of traditional energy sources like coal and natural gas. Studies have consistently shown that solar power generation emits considerably less greenhouse gases over its entire lifecycle, from manufacturing to decommissioning. For example, a typical coal-fired power plant emits several hundred grams of CO2 per kilowatt-hour (kWh) of electricity generated, while the carbon footprint of solar power is usually measured in tens of grams of CO2e (carbon dioxide equivalent) per kWh, representing a substantial reduction in emissions. This difference stems from the fact that solar panels do not directly burn fossil fuels to produce electricity.
Sustainability Aspects of Solar Panel Technology
Solar panel technology contributes to sustainability in several key ways. Firstly, it harnesses a virtually inexhaustible resource – sunlight – to generate electricity. This reduces our reliance on finite fossil fuels and helps mitigate climate change. Secondly, solar power is a decentralized energy source, meaning that solar panels can be installed on rooftops, in fields, and other locations, reducing the need for large-scale centralized power plants and their associated environmental impacts. Thirdly, ongoing research and development are focused on improving the efficiency, durability, and recyclability of solar panels, further enhancing their sustainability profile. For instance, advancements in materials science are leading to the development of more efficient and environmentally friendly solar cells, and recycling programs are emerging to recover valuable materials from end-of-life panels.
Arguments for Solar Panels as a Sustainable Energy Source
The use of solar panels strongly aligns with the principles of sustainable development. They provide a clean, renewable energy source, reducing greenhouse gas emissions and mitigating the effects of climate change. Their decentralized nature reduces the environmental impact associated with large-scale energy infrastructure. Furthermore, the continuous improvements in solar panel technology, including increased efficiency and recyclability, further strengthen their position as a sustainable energy solution. The transition to solar energy contributes to a more resilient and environmentally responsible energy system, promoting long-term sustainability and mitigating the risks associated with fossil fuel dependence. The widespread adoption of solar power represents a significant step towards a cleaner, healthier, and more sustainable future.
Case Studies of Solar Panel Performance in Different Climates
Solar panel performance varies significantly depending on geographical location and prevailing weather conditions. Understanding these variations is crucial for optimizing system design and managing expectations regarding energy production. Analyzing case studies from diverse climates helps illustrate the adaptability and resilience of solar technology in different environments.
This section presents several case studies demonstrating the successful implementation of solar power systems in regions characterized by high rainfall or significant cloud cover. The data provided illustrates the real-world energy production achievable under various climatic conditions, highlighting both successes and challenges encountered.
Case Study Data: Solar Panel Performance in Diverse Climates
The following table summarizes the performance of solar panel installations across different geographical locations, showcasing the variability in energy output based on climate. It’s important to note that these figures represent averages and actual performance can fluctuate due to factors such as seasonal changes, panel orientation, and system maintenance.
Location | Climate | Average Solar Output (kWh/kWp/year) | Challenges |
---|---|---|---|
Seattle, Washington, USA | Temperate, Rainy, Cloudy | 1200-1400 | Reduced sunlight hours, frequent cloud cover necessitate larger system sizes to meet energy demands. System design must account for potential shading from trees and buildings. |
Munich, Germany | Temperate, variable cloud cover | 1000-1200 | Seasonal variations in sunlight availability, requiring careful consideration of panel tilt and orientation to maximize winter production. Snow accumulation can also impact performance. |
Vancouver, British Columbia, Canada | Temperate, rainy, significant cloud cover | 1100-1300 | Similar to Seattle, reduced sunlight hours require larger systems. The challenge is mitigated by using high-efficiency panels and optimizing system design for low-light conditions. |
London, England | Temperate, often cloudy | 900-1100 | Lower solar irradiance compared to sunnier climates requires careful system sizing and potentially energy storage solutions to ensure reliable power supply. |
The success of these installations hinges on several factors. Careful site assessment, considering factors such as shading and orientation, is paramount. Selecting high-efficiency panels designed for low-light conditions is crucial in areas with frequent cloud cover. In some cases, incorporating energy storage solutions, such as batteries, can help mitigate the impact of intermittent sunlight and ensure a consistent power supply.
Factors Contributing to Success and Failure
Successful solar installations in climates with less direct sunlight often involve a combination of strategic planning and advanced technologies. For example, using panels with higher efficiency ratings can compensate for reduced sunlight hours. Optimizing the angle and orientation of the panels to maximize sunlight capture throughout the year is also essential. In contrast, failures often stem from insufficient system sizing, poor site selection (leading to shading), or neglecting the impact of local climate conditions on system performance. Regular maintenance and cleaning are also crucial for maintaining optimal efficiency.
Technological Advancements in Solar Panel Technology
The quest for improved solar energy efficiency, particularly in challenging weather conditions, has driven significant advancements in solar panel technology. Recent innovations focus on enhancing light absorption, improving energy conversion rates, and mitigating the negative effects of rain, cloud cover, and low-light conditions. These advancements utilize new materials, refined designs, and sophisticated manufacturing techniques.
Several key areas have seen substantial progress. These include the development of advanced materials with broader light absorption capabilities, the optimization of panel designs to maximize light capture even at oblique angles, and the integration of smart technologies for real-time performance monitoring and adjustment. The overall goal is to create more robust and efficient solar panels capable of consistently generating power regardless of weather fluctuations.
Perovskite Solar Cells and Tandem Cells
Perovskite solar cells are a rapidly developing technology showing immense promise for low-light performance. Unlike traditional silicon-based cells, perovskites exhibit a wider range of light absorption, making them particularly effective in cloudy or diffused light conditions. Furthermore, the combination of perovskite and silicon in tandem cells creates a synergistic effect, boosting overall efficiency significantly. This technology has the potential to surpass the efficiency limits of traditional silicon solar cells, especially in low-light environments. For example, some research has demonstrated tandem cells achieving efficiencies exceeding 30%, a significant jump from the typical 18-22% of conventional silicon cells. The integration of perovskite technology into commercially available panels is still in its early stages, but rapid advancements suggest widespread adoption in the near future.
Improved Anti-Reflective Coatings and Textured Surfaces
Minimizing light reflection is crucial for maximizing energy capture. Recent advancements in anti-reflective coatings significantly reduce light loss from the panel’s surface. These coatings often incorporate nanostructures or specific materials that minimize the reflection of incident light across a wider range of wavelengths. Additionally, textured surfaces are being developed to scatter incident light, increasing the probability of photons being absorbed by the active material within the cell. This is especially beneficial in low-light conditions, where every photon counts. For instance, textured surfaces can improve light absorption by up to 10%, leading to a noticeable increase in energy output even on cloudy days.
Bifacial Solar Panels
Bifacial solar panels are designed to absorb light from both the front and back surfaces. This feature significantly enhances energy production, particularly in environments with high albedo (reflectivity) such as snow-covered ground or bright surfaces. By capturing light reflected from the ground, bifacial panels can increase energy yield by 10-30% compared to traditional monofacial panels. This design is particularly advantageous in locations with frequent cloud cover, as reflected light can supplement direct sunlight, mitigating the impact of reduced solar irradiance.
Timeline of Key Innovations
A timeline highlighting key innovations in solar panel technology demonstrates the rapid pace of advancements and their impact on performance in various weather conditions:
Year | Innovation | Impact |
---|---|---|
1954 | First silicon solar cell | Foundation of modern solar technology; low efficiency. |
1970s-1980s | Improvements in silicon wafer processing | Gradual increase in efficiency. |
1990s-2000s | Development of thin-film solar cells | Reduced material costs, but lower efficiency than silicon. |
2010s-Present | Perovskite solar cells, tandem cells, improved anti-reflective coatings, bifacial designs | Significant increase in efficiency, especially in low-light conditions. |
FAQ Overview
Do solar panels get damaged by hail?
While most solar panels are designed to withstand hail, extremely large or frequent hailstorms can cause damage. Check your warranty and consider supplemental protection.
How often should I clean my solar panels?
The frequency depends on your location’s climate. In areas with frequent rain, cleaning may be less necessary. However, a yearly cleaning is generally recommended.
Can snow significantly reduce solar panel output?
Yes, snow accumulation can significantly reduce or completely block sunlight from reaching the panels. Regular snow removal is important in snowy climates.
Are solar panels effective in all climates?
Solar panels are effective in various climates, but output varies depending on sunlight availability. Areas with consistent cloud cover will produce less energy than those with abundant sunshine.